The Fallacy of Effective Velocity: Perception Can’t Be Quantified
By Ken Cherryhomes ©2025
Much of what Perry Husband hypothesizes in respect to swing biomechanics, I agree with. However, I struggle with the validity of his effective velocity theory. “Effective velocity” (eV) has been promoted as a framework for understanding pitch deception. The idea is simple but flawed: pitches at different locations in the zone “feel” faster or slower than their actual radar gun readings, and this altered perception changes hitter behavior. A 90 mph fastball up in the zone, for example, might be treated as a 94 mph pitch due to reduced reaction time. It’s an intuitive story—but it’s not a measured one.
That’s because eV models don’t actually measure anything. They rely on fabricated multipliers, assigning higher “effective speeds” to certain zones based on assumptions about swing urgency. These multipliers—often ranging from +0 to +4—are not derived from time-of-flight data, swing mechanics, or perceptual studies. They are approximations meant to simulate difficulty, not quantify it.
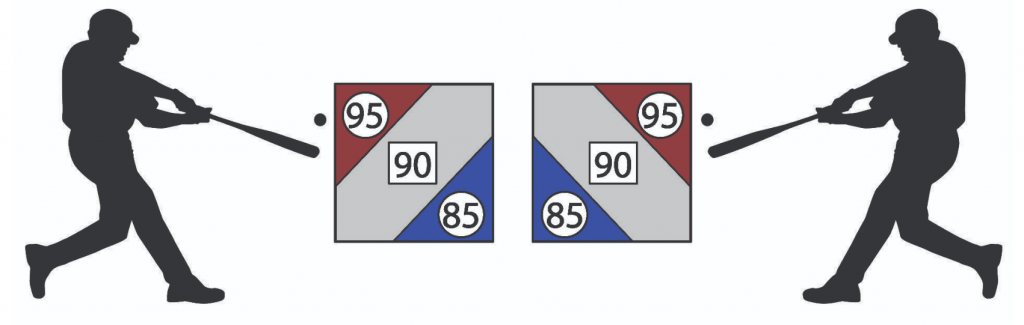
This graphic—originally published by Perry Husband—visually represents the core concept behind effective velocity (eV). The diagram overlays a strike zone divided into three diagonal bands, each labeled with a different “effective speed”:
- Red Zone (Top-Inside): Said to “play” +5 mph faster than the radar speed
- Gray Zone (Middle): Matches the actual pitch speed (e.g., 90 mph)
- Blue Zone (Low-Away): Said to “play” –5 mph slower than radar speed
The implication is that location alone alters how fast a pitch “feels” to a hitter, regardless of radar-measured velocity. Under this model, a 90 mph pitch located up and in is treated as if it’s 95 mph, while the same pitch down and away is perceived as 85 mph. This perceptual difference, eV theory argues, affects swing timing and decision-making.
Although the version shown here uses simple round numbers for clarity, Husband asserts that his system uses more granular values in practice. However, the specific methodology for deriving these adjustments remains unclear and unvalidated.
But when pitch velocity is broken down across strike zone locations—based on known distances, time of flight, and measured swing durations—the results tell a different story.
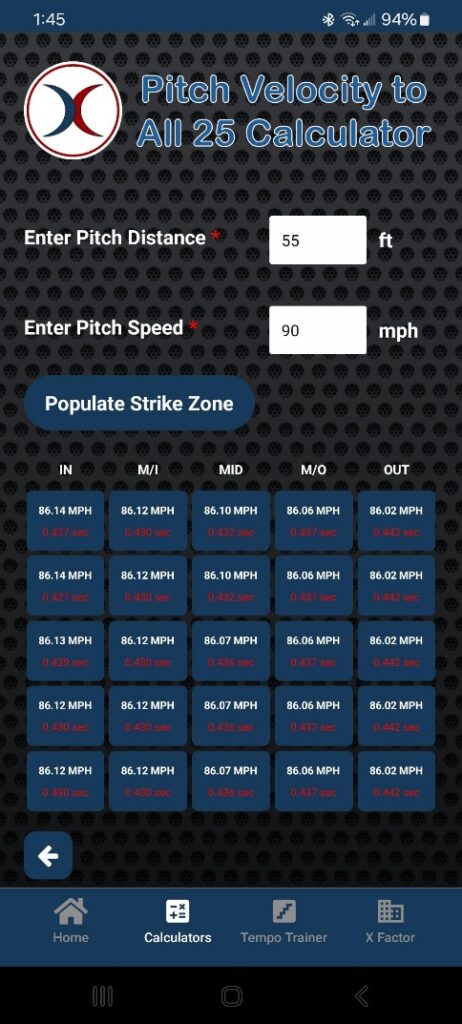
The actual time differences between, say, an inside pitch and an outside pitch, or a low pitch versus a high one, are real but minimal—often within 6–10 milliseconds. These deltas exist, but they don’t justify the dramatic behavioral adjustments we see in hitters.
Why then do batters swing early on outside pitches and late on inside ones?
Because urgency isn’t governed by time alone—it’s governed by perceived threat and outcome risk. The inside pitch threatens bodily space and has limited swing space and spray options, requiring forward contact; delay and hesitation can result in getting jammed. The outside pitch, by contrast, must be hit later in the pitch’s trajectory but feels like it’s getting away due to its increased lateral proximity (distance) from the hitter and its required deeper contact depth, prompting hitters to overreact. These reactions are proprioceptive and outcome-driven, not necessarily reflections of time compression.
This spatially-driven urgency is observable even in slow pitch softball, where the time window is generous and pitch speed is not a constraint. Yet hitters still tend to be late on inside pitches and early on outside pitches—the same failure patterns seen in high-speed environments. These are not the result of compressed reaction time, but of how the pitch location interacts with perceived swing space and proximity. Alongside mistimed swings, we also see clear postural adjustments: hitters physically open up to create room on inside pitches, and hinge forward to reach outside, showing that urgency and swing behavior are governed by spatial intrusion and perceived collision difficulty, not pitch velocity.
In this light, “effective velocity” collapses. It fails to account for the fact that swing decisions are shaped by the hitter’s attempt to avoid spatial inefficiency—not by phantom increases in pitch speed.
Any timing model that aims to be valid must deal with the actual constraints a hitter faces: time over distance, swing arc geometry, and contact point depth. These are measurable. “Perceived speed” is not.
Let’s go over Husband’s theoretical model.
The Hypothesis: By throwing pitches in the warm up round at the same basic reactionary speed, the hitters would be ‘geared’ to that speed. Then throwing the same speed, but moving pitches around the strike zone strategically, hitters should be early and late, proving they were focused at one basic speed and location changes added or subtracted reaction time. By moving the ball around the strike zone, this adds or subtracts EvMPH with no radar speed changes more than 1 MPH. Note that the hitter doesn’t know anything about the test at these lower speeds other than they have to swing at all strikes. The goal was to have hitters in a ‘reactionary mindset’ with no hints as to the order or location, simply testing reactionary skills at a very basic level. ~ Perry Husband
Based on his model, pitches located in the strike zone are perceived to be up to 4-5 mph faster or slower than a pitch down the middle dependent upon where an estimated point of contact with the pitch is located.
This is where the theory reveals itself as just that—a theory grounded in subjective assumptions about how velocity and proximity are perceived. Here’s a breakdown that exposes the flaws:
- Swing space is not uniform; it’s a dynamic zone shaped by proximity, perceived threat (whether physical, cognitive, or outcome-based), and the batter’s internalized comfort with range of motion.
- Inside pitches, though arriving earlier, must be struck later in the arc—farther forward—requiring earlier swing initiation. The danger here is visceral: the pitch encroaches on personal space, compressing swing freedom. Ironically, hitters often delay—not due to lack of time, but because of hesitation, uncertainty, or discomfort—resulting in late swings.
- Outside pitches, though arriving later, must be struck deeper—earlier in the arc—which allows for later swing initiation. But the lateral distance creates the illusion of the pitch “getting away,” inducing false urgency. The result: premature swings on a pitch that never posed immediate danger.
- Middle pitches land squarely in the hitter’s proprioceptive comfort zone, requiring minimal compensation—hence, consistent timing success.
- Proprioception governs swing space awareness: outside pitches enter cleanly but remain outside the body’s collision comfort zone, while inside pitches invade it—demanding engagement in a region the body doesn’t instinctively favor. This contrast triggers asymmetrical urgency and divergent swing behaviors.
In summary: the common failure modes are reversed. Hitters tend to be late on inside pitches, failing to launch early enough due to perceived danger and spatial intrusion, and early on outside pitches, initiating too soon because the ball—farther away and requiring deeper contact—feels like it’s escaping. This mismatch between perceived urgency and actual timing demand explains the systemic error patterns across pitch locations.
Empirical Breakdown of Pitch Timing and Swing Windows
This is where theory meets reality: we turn from perceived urgency to measured swing times and pitch velocities mapped across all 25 strike zone locations.
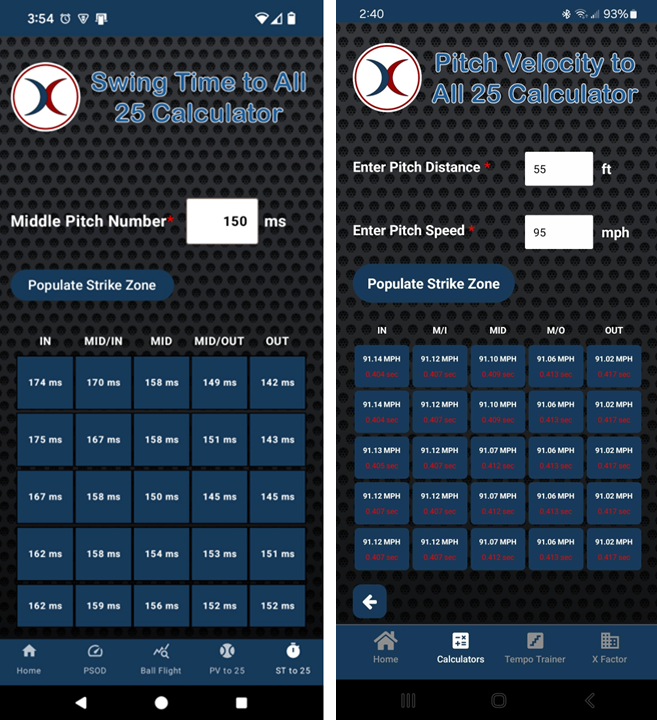
But under the eV theory:
- That same high fastball is arbitrarily said to “feel like” 95 or 96 mph, and the low-away pitch like 89–90 mph in effective velocity, despite average velocities across the zone differing by only 0.12 mph.
- Swing execution times show just a 22 ms difference between the two extremes.
- “Perceived urgency” is assigned a multiplier—yet this tool makes that guesswork obsolete by replacing it with measurable timing windows.
A swing-time-to-25-locations calculator and a pitch-velocity-to-25-locations calculator (accounting for drag and depth) replace the flawed concept of effective velocity by removing subjectivity from timing analysis. Rather than applying arbitrary multipliers to simulate urgency, we can observe real physics—calculating actual time over distance and individualized swing times to 25 contact points. This allows for precise, objective determination of when a batter must initiate their swing for each pitch location.
It renders the concept of “perceived speed” obsolete by grounding timing demands in measurable constraints, not fabricated adjustments. Simply put: we’re not guessing how fast a pitch feels—we’re measuring how much time the hitter actually has.
And that time—spanning only a few milliseconds across the zone—is not enough to account for the dramatic behavioral differences seen in hitters. If urgency were strictly about time, hitters wouldn’t show such drastic swing deviations across such narrow deltas.
Case Study: Mitigating Perceived Velocity Through Precise Timing and Contact Mapping
Hypothesis (eV model)
Husband proposes that when hitters are “geared” to a single pitch speed—typically through repetition in warm-up rounds—they develop an internal timing expectation. If the pitcher maintains that same radar velocity but varies location, the hitter is said to become confounded. According to the effective velocity (eV) model, shifting pitch location alters perceived timing pressure. A pitch up-and-in is said to “add” mph, while a pitch down-and-away “subtracts” it—even without any real change in speed.
In short, once calibrated to a single speed, moving the pitch across the zone should induce early or late contact errors based solely on location-driven perceptual distortion.
Challenging the Model
In this controlled 4-pitch sequence, the batter was kept unaware of pitch order and instructed only to swing at strikes. The velocity of each pitch remained nearly constant (~91 mph). The sequence was as follows:
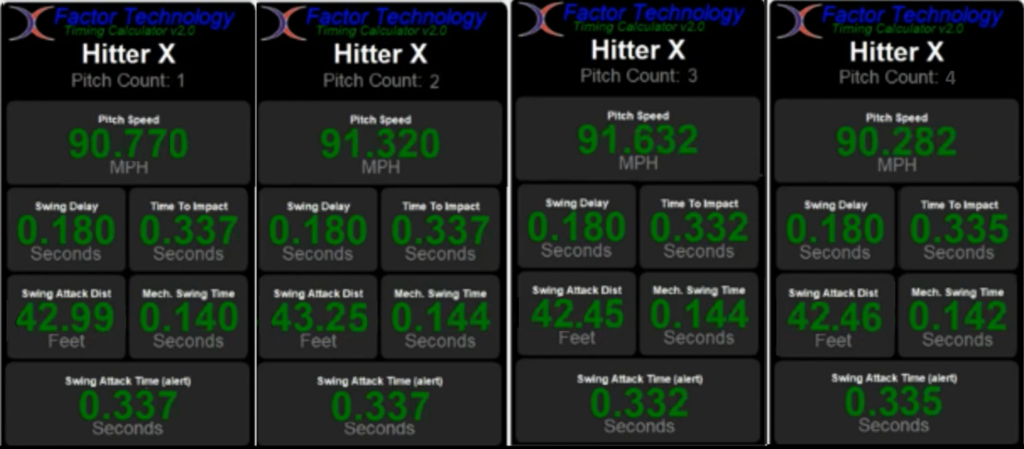
- Inside pitch – establishing the calibration point (location and velocity).
- Second inside pitch – reinforcing spatial and temporal expectation.
- Far outside pitch – maximal spatial disruption.
- Middle pitch – returning to neutral location.
Throughout the sequence, the batter was issued precise swing cues, and proprioceptive timing was calibrated to match known contact depths for each location. Swing initiation (attack time) and Online control for mechanical swing time was tracked live.
Observation
In this four-pitch sequence—two inside, followed by an outside pitch, then a middle pitch—all thrown at roughly the same speed (~91 mph), the batter’s swing initiation was guided by precise Time to Impact (TTI) values, each derived from known spatial/temporal contact points.
Despite the dramatic shift in pitch location, from inside to outside to middle, the batter initiated each swing at the correct time—matching the required TTI at each location with no measurable confusion or overcorrection.
TTI and Swing Attack Times:
- Pitch 1 (Inside): TTI 0.337 sec | Attack Time 0.337 sec
- Pitch 2 (Inside): TTI 0.337 sec | Attack Time 0.337 sec
- Pitch 3 (Outside): TTI 0.332 sec | Attack Time 0.332 sec
- Pitch 4 (Middle): TTI 0.335 sec | Attack Time 0.335 sec
The swing attack times shifted in perfect alignment with the calculated TTI values, despite location moving from one spatial extreme to another.
Mechanical swing times changed slightly due to perception-action and online control variances.
Mechanical Swing Time:
- Pitch 1 (Inside): Swing time: 0.140 sec | Attack Time 0.337 sec
- Pitch 2 (Inside): Swing time: 0.144 sec | Attack Time 0.337 sec
- Pitch 3 (Outside): Swing time: 0.144 sec | Attack Time 0.332 sec
- Pitch 4 (Middle): Swing time: 0.142 sec | Attack Time 0.335 sec
Spatial Variation, Temporal Precision: One Swing, Three Locations
The following three swings represent pitches from the 4-pitch sequence: inside, outside, and middle. Each was thrown at approximately the same velocity (~91 mph), and each was guided by a location-specific TTI cue derived from spatial/temporal mapping.
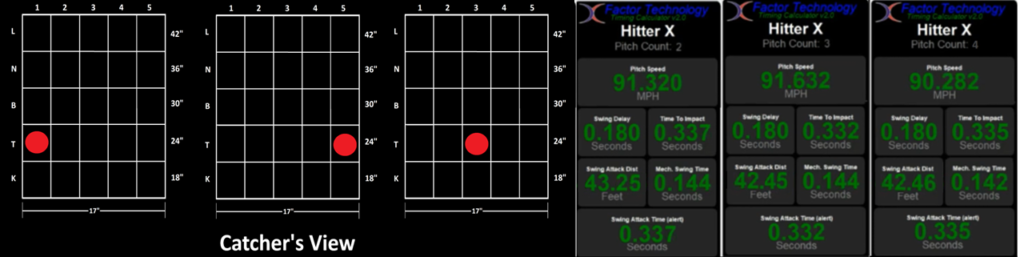
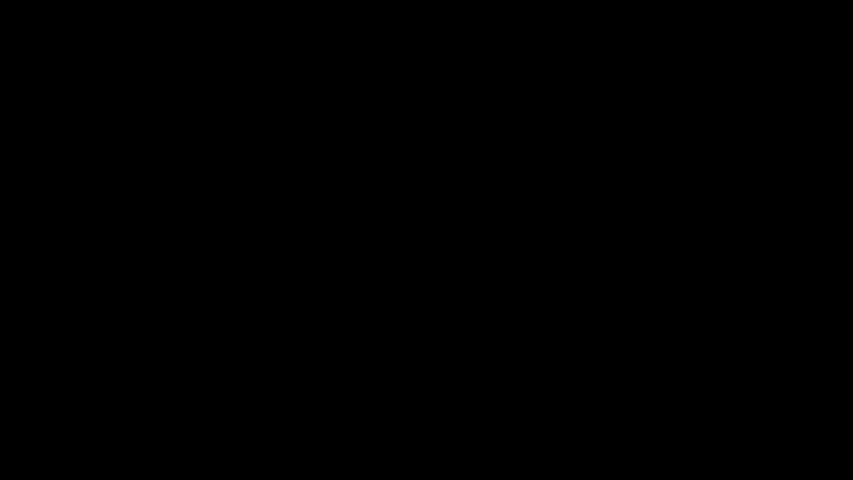
In the aligned GIF shown below, these three swings—when overlaid and synchronized by swing arc—appear indistinguishable in shape and path. Visually, it resembles a single continuous swing striking three separate pitches in different locations.
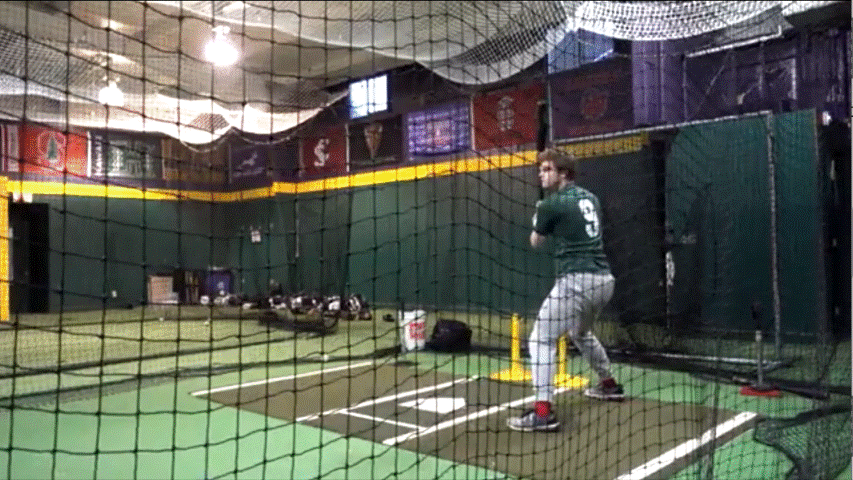
The batter’s posture, contact orientation, and barrel alignment reflected internalized spatial solutions, not recalibrated perceptions of velocity. This was not about perceived speed; it was about solving the geometry of collision.
The “effective velocity” model presumes perception is fooled by spatial variation. But the batter in this sequence shows no confusion—only coordinated, context-dependent swing execution. And it’s measurable. The margin of error between pitches was under 0.005 seconds. If the hitter was early or late, it wasn’t because the pitch “felt” 4 mph faster or slower. It was because collision strategy and spatial pressure vary by location, not because the brain misjudged speed.
There were no compensatory swing path changes, no visible re-aiming, and no perceptual hesitation—just a precise shift in timing that can be internalized.
The bat path remains constant. Only when the swing intersects the pitch differs—not how the swing moves through space.
This directly contradicts the eV model’s claim that shifting pitch location—even at a constant speed—should induce mistimed swings due to perceived changes in velocity. What we observe instead is location-specific precision, with swing timing guided by spatial awareness and calibrated contact depth—not perceptual misjudgment of speed.
Conclusion
The eV framework misdiagnoses the source of swing urgency. It attributes timing pressure to fractional differences in pitch arrival time and swing execution time across the strike zone, but the real constraint is spatial and outcome-driven. Batters swing early or late not because of how fast a pitch feels, but because of biomechanical and perceptual factors tied to collision efficiency and the need to avoid failure.
This performance exposes that disconnect. Where the eV model predicts timing disruption due to perceived velocity shifts, the hitter demonstrated precise, location-specific solutions—guided by proprioception, spatial awareness, and calibrated contact points.
Swing timing was not shaped by deception or illusion. It was shaped by geometry, intent, and measurable constraints. In that light, effective velocity becomes unnecessary—a narrative framework attempting to explain a problem that can already be solved with precision.